ctDNA: The Most Important Cancer Liquid Biopsy Biomarker?
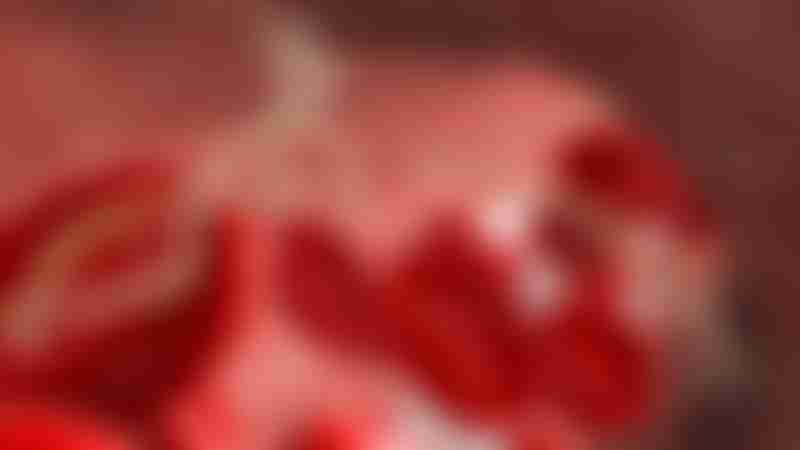
Source: Shutterstock.
Exploration and research into different novel biomarkers for cancer have been ramping up in recent decades, owing largely to the game-changing applications of cancer biomarkers (sometimes called tumor markers) in detection, diagnosis, prognosis and treatment.
Circulating tumor DNA (ctDNA) is an emerging colorectal cancer biomarker that has been touted to prove particularly useful not only in treatment management, but also in cancer recurrence monitoring. Its explored applications have mainly been through liquid biopsy — a sampling and analysis of bodily fluids such as blood for cancer markers.
Is ctDNA the most important cancer biomarker to date, and how does it impact the current liquid biopsy standard?
What is ctDNA?
ctDNA, as the name suggests, is DNA that circulates throughout the body. It belongs to a subset of DNA known as cell-free DNA (cfDNA), which is characterized by DNA that is not enclosed within a cell. With tumors, cells are often shed and released into vascular systems such as the bloodstream or lymphatic system, and when these cells undergo apoptosis or programmed cell death, the DNA is released into these body fluids.
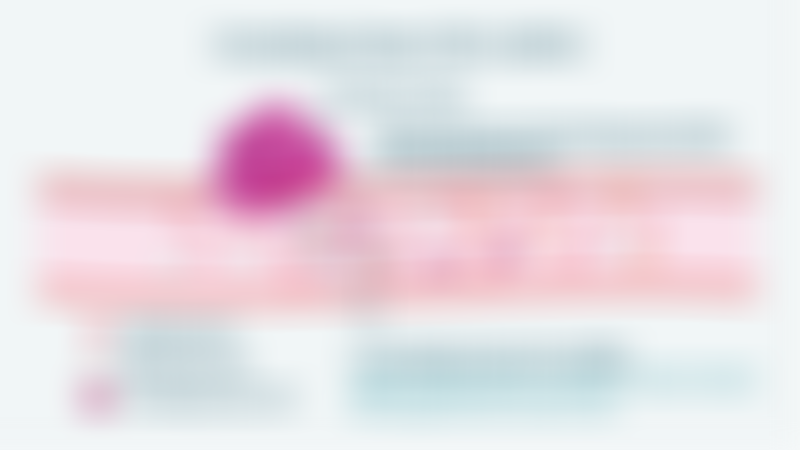
How ctDNA is found in the blood.
Adapted from: Shutterstock.
More specifically, the three main sources of ctDNA are apoptotic or necrotic tumor cells, active tumor cells and circulating tumor cells. This confers ctDNA a special advantage. The genetic information contained within ctDNA found in the peripheral blood is identical to the tumor it originates from, and successful detection and analysis of ctDNA will be able to present a real-time profile of the cancer at different intervals such as before and after treatment.
How ctDNA is detected
The crux of ctDNA’s utility in liquid biopsy lies in detecting the genetic mutations associated with colorectal cancer. However, ctDNA is found in very small quantities in peripheral blood, which could make it potentially difficult to obtain. To that end, there are several employable tools, each with their advantages and disadvantages based on sensitivity and comprehensiveness.
The different detection technologies can be broadly classified into three categories: single-locus or multiplexed assays, targeted sequencing approaches and genome-wide analysis.
Single-locus or multiplexed assays are highly sensitive and inexpensive tests that are able to rapidly detect specific point mutations, copy number variations, short indels and gene fusions. The assays do not require high concentrations of ctDNA, however, they can only be used to monitor for a small number of known mutations.
Examples: Microfluidic or allele-specific PCR; allele-specific quantitative PCR; droplet digital PCR; beads, emulsion, amplification and magnetics (BEAMing)
Targeted sequencing approaches look at multiple loci on the genome and monitor for de novo resistance mutations and clonal evolution in response to therapy. This approach can also be used to monitor the sensitivity for disease burden by testing multiple loci in a single assay. However, monitoring of multiple loci takes a long time to analyze and is a costly process.
Examples: Amplicon sequencing; Safe-Seq; TAm-Seq; Hybridization capture; CAPP-Seq
Similar to targeted sequencing, genome-wide analysis identifies structural variants in the genome, but instead of looking at specific loci, it scans the entire genome. This allows for detection of chromosomal aberrations and also stratification of patient samples based on disease burden. Nonetheless, the procedure is also expensive and takes a long time to complete.
Example: Whole-exome sequencing
Potential applications of ctDNA
cfDNA, and by inclusion, ctDNA, do not remain long in the bloodstream and are rapidly removed in the kidneys, liver and spleen. Studies have shown that the half-life of cfDNA, or the time it takes for the quantity of cfDNA to halve, takes between 16 minutes to several hours, which is what allows it to be used in real-time cancer profiling.
ctDNA could potentially be applied to early colorectal cancer detection by analyzing liquid biopsy samples for methylated ctDNA in combination with other tumor markers. One particular study showed that ctDNA methylation marker cg10673833 was able to yield, with high sensitivity and specificity, the detection of colorectal cancer in nearly 1,500 participants.
However, ctDNA levels are also found to be elevated in other medical conditions such as myocardial infarctions, infection and inflammatory diseases. In addition, benign lesions that have the same mutations as cancer cells may also release cfDNA into the bloodstream. Examining the length of the cfDNA fragments can overcome this non-specificity. cfDNA in healthy individuals have been shown to exhibit distinct patterns in fragment length. On the flip side, cfDNA fragment length in cancer patients is irregular. Nonetheless, further study is required to fully utilize ctDNA in early colorectal cancer detection.
ctDNA may also prove useful in cancer prognostics, as studies have found that levels of ctDNA correlate with worse patient survival regardless of tumor stage. In post-diagnosis settings, ctDNA can also be used to identify a patient’s medical resistance by examining the genetic mutations found in the ctDNA. These include mutations in the KRAS, BRAF and NRAS genes, each resulting in resistance to epidermal growth factor receptor (EGFR) inhibitors like cetuximab and panitumumab.
A work-in-progress
ctDNA, together with liquid biopsy, holds several advantages over conventional tissue biopsy, which can only capture static information about the cancer at the time of sampling, and is a more invasive sampling method even if done laparoscopically. However, a liquid biopsy alone is still unable to give the full picture; it lacks a histological and cellular profile of the cancer, which can only be found by direct examination of the tumor tissue collected through conventional biopsy.
With that having been said, liquid biopsy shouldn’t be seen as a direct replacement for tissue biopsy, but instead as complementary to it in order to provide more holistic and comprehensive analyses of the cancer.
As it stands, more work is still needed to not only standardize detection methods for cfDNA and ctDNA, but also to further optimize ctDNA analysis and evaluation due to differential correlations between ctDNA and clinicopathological factors. Nonetheless, a growing repertoire of non-invasive screening, detection and diagnosis methods can go a long way in improving patient compliance and earlier colorectal cancer treatment.